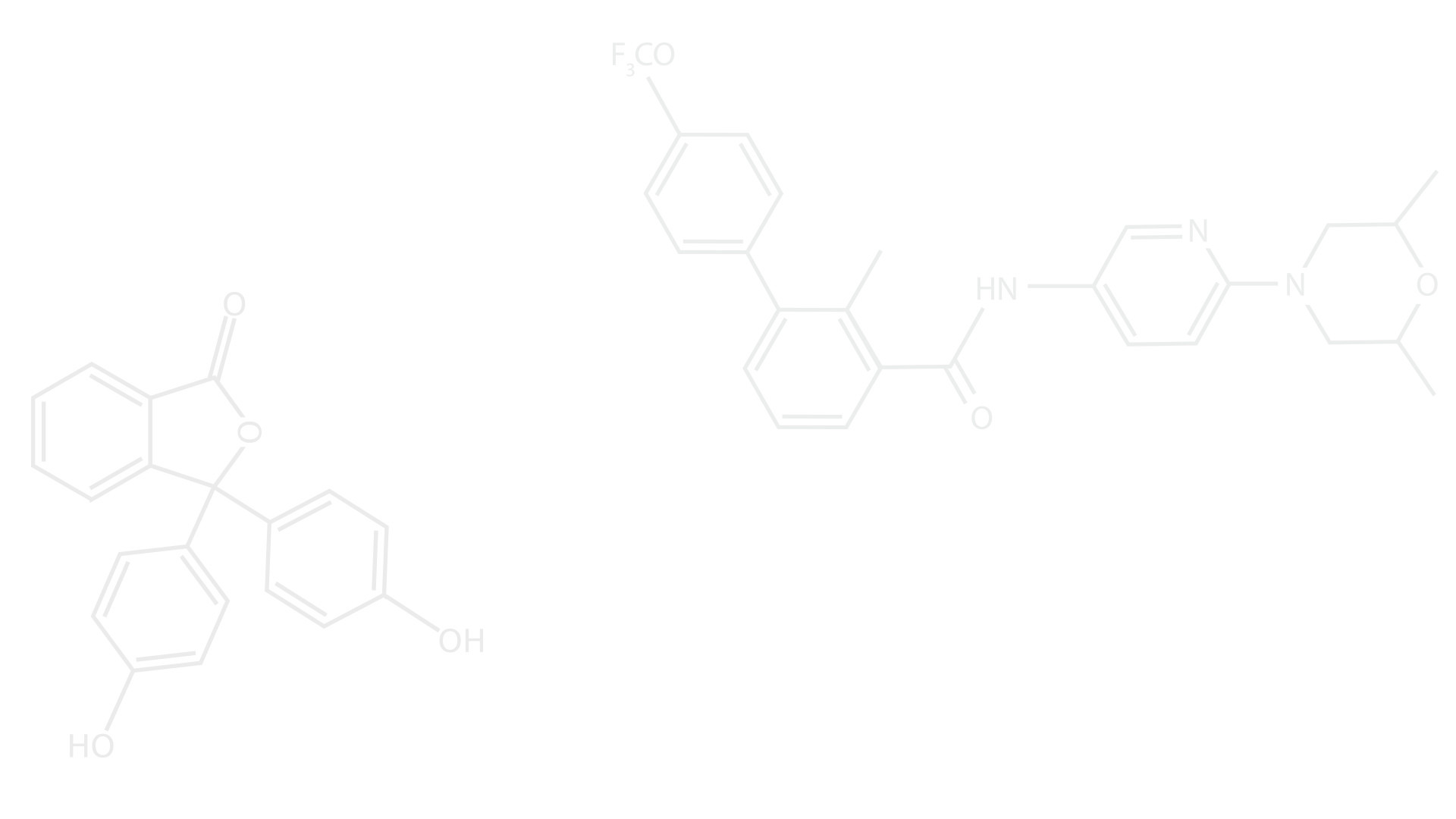
What we do:
1) DNA-encoded chemical library (DEL) and its applications.
Fig. 1. Selection of DNA-encoded chemical library (DEL) against protein targets for ligand discovery.
Originally proposed by Brenner and Lerner (1), DEL has emerged as a powerful high-throughput screening technology (2-4). In a DEL, each compound is conjugated with a DNA tag encoding its chemical structure. All library compounds are screened against the target in one pot based on binding affinity. Selected binders are decoded by PCR amplification and next-generation sequencing (NGS) to read the barcodes (Fig. 1). DELs can contain hundreds of millions to many billions of compounds and screening can be rapidly performed in a few hours. In the past decade, DELs have been widely adopted by the pharmaceutical industry in many drug discovery programs (5-8).
We are one of the academic groups with an established track record in this technology and we focus on bridging the gap between technology development and applications. Previously, we have developed a “Universal Template” approach, which eliminated the need for complicated codon design and the entire library could be prepared with just one DNA template. Recently, we introduced DNA encoding to traditional dynamic combinatorial library (DCL) and developed a series of DNA-encoded dynamic library (DEDL) methods, which circumvented the long-lasting problem of low chemical diversity of DCL and enabled efficient inhibitor discovery for protein targets. We also developed a series of methods to enable ligand DEL screening against the protein targets previously intractable to DELs. Typically, DELs are selected against purified proteins immobilized on a matrix; however, many proteins are not suitable for modification and immobilization. To address this limitation, we developed crosslinking-based methods that can interrogate proteins directly in buffer or in cell lysates. Moreover, membrane proteins play a myriad of important roles in cells and are implicated in many human diseases; however, ligand discovery for membrane proteins is highly challenging, since a natural cell membrane environment is often necessary to maintain protein structure and functions. Recently, we have developed a method that can perform large-scale DEL screening against endogenous membrane proteins on live cells without over-expression or any genetic manipulation. This method is expected to expedite drug discovery for many important membrane protein drug targets (e.g. GPCR, ion channels) previously intractable to high-throughput screening.
Current research on DEL:
We consider that DEL should not be limited to just a binding assay, but could also be used to explore biological functions. For example, we initiated a project to use DNA to encode the macrocyclization, rather than macrocycle structure, to identify macrocycle ligands. We are also using DEL to identify functional modulators for membrane proteins, e.g. activators/inhibitors for receptor tyrosine kinases (targeting the extracellular domain), allosteric agonists for GPCRs, antagonists for immune-checkpoint proteins, etc. We are extending the application of DNA encoding to explore protein-protein interaction systems, such as the identification and profiling of the extracellular ligands for membrane proteins (integrins and TNF receptors). The identification of their binding partners in the extracellular matrix will provide valuable insights into their biological functions. In addition, we are collaborating with other research groups to develop novel theranostic use of DEL for the chemo-/radio-therapeutics of malignant cancers and a new type of multivalent DEL for interrogating protein complexes with multiple, distal binding pockets.
2) Protein labeling and profiling.
Fig. 2. a) Scheme for the DNA-Programmed Affinity Labeling (DPAL). b) Using DPAL to profile HDAC complexes.
We aim to develop new methods to enable more specific, sensitive, and versatile labeling of proteins. Previously, we developed a protein labeling and profiling method named DNA-Programmed Affinity Labeling (DPAL; Fig. 2a). This method employs a unique dual-probe system where the functions of target binding and labeling are separated and programmed by DNA-based binding probe and capture probe. DPAL is able to present multiple probe configurations without the need to synthesize multiple affinity probes. We have applied DPAL in target identification of small molecules and labeling of transcription factors and epigenetic mark readers. Recently, we made a major advancement by using DPAL to profile HDAC protein complexes (Fig. 2b). Notably, this method identified many indirect binding proteins in the complex, which were inaccessible to traditional affinity probes due to the distance, and it also revealed new biological functions for HDAC-associated proteins. This work has provided a simple and broadly applicable method to characterize protein complexes. Other researchers have also used DPAL, or approaches with similar concept, in other biological applications, such as site-specific antibody labeling and aptamer-templated protein conjugation.
Fig. 3: Protein labeling with a hidden aldehyde functionality.
Current research on protein labeling and profiling:
On the one hand, we aim to further expand the utility of DPAL to more complex biological systems, such as studying the membrane protein complexes. On the other hand, we are also working on new protein labeling method using non-DNA-based probes. Chemical modification of proteins is a powerful tool to realize a broad range of biological applications. We recently reported an approach to install an aldehyde group to proteins using an affinity probe with a “hidden” functionality that is only exposed upon target binding (Fig. 3). Although still in the early stage, it may represent a new concept in protein labeling. We will further develop the methodology and explore its potential in enabling exciting biological applications such as real-time imaging and conditional protein labeling.
3) Target identification (target ID) and mechanism study of bioactive compounds.
Fig. 4: Our previous target ID projects. .
We are interested in studying bioactive small molecules whose biological activity or therapeutic efficacy is already known but with unknown target and mechanism, especially natural products and drug molecules. We aimed to identify the targets and elucidate the underlying mechanism, which could lead to exciting opportunities for drug discovery and fundamental biology. Our previous target ID work includes (Fig. 4): i) Terazosin is an approved drug to treat hypertension, and it has a well-established target α1-adrenergic receptor. Through a collaboration with two biology research groups, we discovered that terazosin had a new target phosphoglycerate kinase 1 (pgk-1), which is responsible for its novel anti-apoptosis activity in cells and strong protective effects in mouse models of sepsis and stroke. This work revealed new biological functions for pgk-1 other than glycolysis and, and it may facilitate the repurposing of terazosin as a novel anti-sepsis/stroke drug, considering its well-established safety profiles in humans. ii) Alisertib is a highly specific aurora kinase A inhibitor and an anti-cancer drug candidate in late-stage clinical trials. We have identified two new targets of Alisertib, p38 MAPK and laminin receptor, and also studied the mechanisms. This study revealed new mode of action of Alisertib and provided molecular basis for its medicinal optimization. iii) Matrine is a natural alkaloid and a major active component in Sophora flavescens, which has been used in traditional Chinese medicine (TCM) for centuries but with unknown target. We identified Annexin A2 as the target of matrine responsible for its anti-migration activity in cancer cells (Chem. Comm. 2017). This work may accelerate the development of matrine as an anti-cancer drug, rather than just a component in TCM regimens.
Current research on target identification.
i) Motuporamine C (MotC) is a natural alkaloid from sea sponge with potent anti-migration/metastasis activities but very low cytotoxicity in cancer cells. We have identified the target of MotC and also validated its functional relevance. Interestingly, this target protein is very poorly characterized in mammalian cells with less than a dozen literature reports, but its bacterial ortholog is known to catalyze biochemical reactions that do not exist in mammalian cells. Currently, we are investigating the underlying mechanism, which might lead to the discovery of a new regulator of cell migration.
ii) Panax notoginseng saponins. Panax notoginseng (PN) is a species of the genus Panax, commonly called Chinese ginseng or San Qi (三七) in Chinese. PN is one of the most well-known TCMs and has been traditionally used to stop bleeding, relieve pain, reduce swelling and blood pressure, and relieve symptoms from strokes and heart attacks. It is also used to improve energy and ability to exercise and for osteoarthritis and rheumatoid arthritis. It is generally believed that the five major Panax notoginsenosides (三七總皂苷), also called PN saponins, are responsible for the medicinal effects of PN. However, despite the rich history in TCM and the wide clinical uses in modern medicine, none of the targets of the PN saponins is known, let alone the molecule mechanisms underlying their therapeutic effects. It would be highly revealing to compare the targets identified for each of the PN saponins.
iii) Active components in Scutellaria baicalensis: Scutellaria baicalensis (ScB), commonly called Chinese skullcap (黃芩) is one of the 50 fundamental herbs in TCM. Traditionally, ScB is considered cold in nature and used to treat a myriad of diseases, including respiratory infections, fever, headache, sores or swelling, gastrointestinal infections, hepatitis, inflammations, psychiatric conditions, and even HIV/AIDS. Modern TCM also includes ScB to treat prostate cancer and arthritis. Extracts of ScB have been found to have protective effects in cells under oxidative stress. Several major components from ScB have been isolated and characterized, but their molecular targets and mode of actions have yet to be explored. We are using the DPAL method to conduct target ID study for these compounds.
References:
1. Brenner, S.; Lerner, R. A., Proc. Nat. Acad. Sci. USA 1992, 89 (12), 5381-3.
2. Goodnow, R. A., Jr.; Dumelin, C. E.; Keefe, A. D., Nat. Rev. Drug Discov. 2017, 16 (2), 131-147.
3. Neri, D.; Lerner, R. A., Annu. Rev. Biochem. 2018, 87, 479-502.
4. Dickson, P.; Kodadek, T., Org. Biomol. Chem. 2019, 17 (19), 4676-4688.
5. Zimmermann, G.; Neri, D., Drug Discov. Today 2016, 21 (11), 1828-1834.
6. Mullard, A., Nature 2016, 530 (7590), 367-9.
7. Gura, T., Science 2015, 350 (6265), 1139-40.
8. Zhao, G.; Huang, Y.; Zhou, Y.; Li, Y.; Li, X., Expert Opin. Drug Discov. 2019, 14, 735-753.



